We will focus more specifically on the organic compounds that incorporate carbonyl groups: aldehydes and ketones.
Key Terms
- Aldehyde
- Formyl group
- Ketone
- Hydrogen bonding
- Hydration
- Hydrate
Objectives
- Identify IUPAC names for simple aldehydes and ketones
- Describe the boiling point and solubility characteristics of aldehydes and ketones relative to those of alkanes and alcohols
- Characterize the process of nucleophilic addition to the carbonyl group
The carbonyl group is shown below in the context of synthesizing alcohols. This functional group is the key component of aldehydes and ketones, which we will discuss here.
Nomenclature for Aldehydes and Ketones
Aldehydes and ketones are structurally similar; the only difference is that for an aldehyde, the carbonyl group has at most one substituent alkyl group, whereas the carbonyl group in a ketone has two. Several examples of aldehydes and ketones are depicted below.
Aldehydes are named by replacing the -e ending of an alkane with -al (similarly to the use of -ol in alcohols). The base molecule is the longest carbon chain ending with the carbonyl group. Furthermore, the carbon atom in the carbonyl group is assumed to be carbon 1, so a number is not needed in the IUPAC name to identify the location of the doubly bonded oxygen atom. If the chain contains two carbonyl groups, one at each end, the correct suffix is -dial (used in the same manner as -diol for compounds with two hydroxyl groups). An example aldehyde is shown below with its IUPAC name.
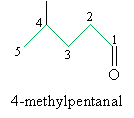
One- and two-carbon aldehydes have common names (one of which you will likely be familiar with) in addition to their systematic names. Both names are acceptable.
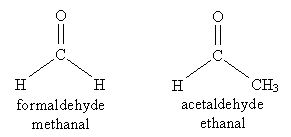
Sometimes, the carbonyl group plus one proton (called a formyl group) must be treated separately for nomenclature purposes, such as when attached to benzene or another ring structure. In such cases, the full base molecule name is followed by the suffix -carbaldehyde, as in the example below. Note that this molecule also has a common name in addition to its systematic name.
For ketones, the indicative suffix is -one. Because the carbonyl group in a ketone can be located anywhere (not just at the end of a carbon chain), a number is required in the name to specify the location of the doubly bonded oxygen atom. An example ketone and its corresponding name is shown below.
Another acceptable (but less preferred) method of naming ketones is similar to that of ethers. The example below illustrates this alternative. (Notice also that the number identifying the location of the carbonyl group can either precede the base name of the carbon chain or follow it.)
When the carbonyl group is part of a ring structure, the oxygen is assumed to be attached to the carbon at position 1. Thus, for instance:
Practice Problem: Name the molecule below.
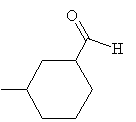
Solution: This molecule is an aldehyde, as the formyl group attached to the cyclohexane ring indicates. To properly name this molecule, we must use the -carbaldehyde suffix. Note the numbering of the carbon atoms in the ring; the IUPAC name is given below the molecule. Note also that because the formyl group is assumed to be at position 1, no number is required in the name to indicate the position of that group.
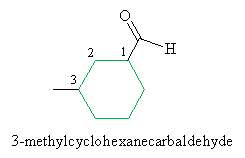
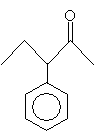
Solution: Number the carbon chain such that the carbonyl group is at the lowest position number.
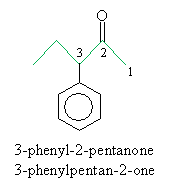
Properties of Aldehydes and Ketones
Remember that van der Waals forces-intermolecular forces that result from dipole moments or induced dipole moments in the participating compounds. A particular type of van der Waals force is hydrogen bonding, which results from dipoles created when hydrogen is bonded to an electronegative element like oxygen or nitrogen. Because the electronegative element "hogs" the electrons shared in the bond, it has a net negative charge compared with the more positively charged hydrogen atom. Hydrogen bonds can form between, for instance, alcohol molecules; an example of a hydrogen bond is shown as the broken line in the diagram below.
Because hydrogen bonds are relatively strong van der Waals forces, they increase the boiling point of molecules like short-carbon-chain alcohols (because more heat energy is needed to break the hydrogen bonds and form a gas from the liquid state). Alkanes cannot undergo hydrogen bonding, so their boiling points are lower. Aldehydes and ketones fall between alkanes and alcohols, as they have an electronegative oxygen atom, but this atom is not bound to a hydrogen atom. As a result, the carbonyl groups do not undergo the same type of attractive force.
Furthermore, hydrogen bonding allows short-carbon-chain alcohols to be highly soluble in water (water can form intermolecular bonds with these alcohols). Alkanes, on the other hand, cannot form these bonds because they are nonpolar molecules. And since alkanes are less dense than water, they tend to separate into a separate (higher) layer. Aldehydes and ketones likewise fall somewhere between alcohols and alkanes in solubility.
Nucleophilic Addition: Hydration
A carbonyl group can be converted to an alcohol in the presence of water and sodium borohydride.

Because oxygen is relatively electronegative, the carbonyl group is polar, as mentioned above.
Thus, the carbon atom makes a good target for nucleophilic attack (depending in part on the substituent groups attached to the carbon atom). In the presence of water, a carbonyl group can undergo some amount of hydration. The overall hydration reaction is shown below for the example case of formaldehyde. The product of this reaction is called a hydrate.
Hydration can be catalyzed by either an acid or base. For acid-catalyzed hydration in water, the following mechanism takes place. In this case, acetone (dimethyl ketone) is used as the example reactant. In the first step, the hydronium ion (H3O+) donates a proton to the carbonyl oxygen.
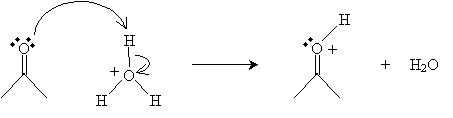
Next, a water molecule attacks the carbon in the carbonyl group.
Finally, a water molecule receives a proton from the cation to form a diol (hydrate).
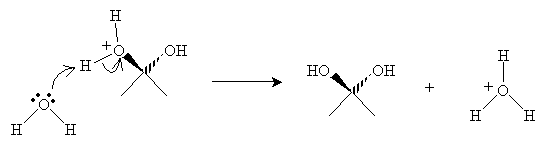
Similarly, hydration can take place in the presence of a base. The reaction mechanism begins with a hydroxide ion attacking the carbon in the carbonyl group.
Finally, water transfers a proton to the oxygen anion, yielding the hydrate and a new hydroxide ion.
The extent to which an aldehyde or ketone is hydrated in water depends on the stability of the carbonyl group (electrical characteristics of the molecule) and on crowding of the carbonyl group (steric effects). The more stable (i.e., the lower in energy) the carbonyl group, the less it will undergo hydration. Thus, when the carbonyl group is more highly substituted with alkyl groups, it is less prone to hydration in water. For instance, formaldehyde will undergo significant hydration, whereas acetone undergoes very little.
Likewise, crowding of the carbonyl group hinders nucleophilic attack, meaning that more substitution (and larger substituent groups) on the carbonyl group decrease the amount of hydration.
Practice Problem: Pentanal and 2-pentanone have the same chemical formula: C5H10O. The aldehyde, pentanal, produces more heat when it combusts (burns) than when an equal amount of 2-pentanone combusts. Propose an explanation for why this is so.
Solution: In our discussion of susceptibility to nucleophilic attack, we noted that a molecule with a more highly substituted carbonyl group is more stable than one with a less highly substituted carbonyl group. In this case, the aldehyde-pentanal-has a monosubstituted carbonyl group, whereas the ketone-2-pentanone-has a disubstituted carbonyl group, making it more stable. And because greater stability means lower energy, we generally expect combustion to yield more energy from less stable molecules than from more stable molecules. Therefore, pentanal should yield more heat energy when it combusts than should an equal amount of 2-pentanone.
Practice Problem: Hydrogen cyanide (HCN) forms a cyanohydrin (shown below) when added to acetone. Propose a mechanism for this reaction.
Solution: Hydrogen cyanide is a weak acid that dissociates to a small extent into protons and cyanide ions (CN-). The cyanide ion is a nucleophile that attacks the carbonyl group as shown below.
A hydrogen cyanide molecule then acts as a Lewis acid with the nucleophilic anion created above to form a cyanohydrin and a cyanide ion.
This mechanism is comparable to the base-catalyzed hydration of acetone.